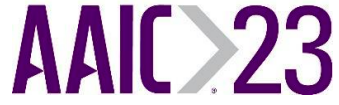
Overview
APOE4:
- Impairs export of toxic fatty acids from human neurons to glia – Femke Feringa
- Induces pro-inflammatory metabolic changes in microglia – Nicholas Devanney
- Prevents microglia from responding to AD pathology and apoptotic neurons – Oleg Butovsky
- Can be switched to APOE2 in mouse models of AD to improve pathology – Lesley Golden, Lance Johnson
Presentation Summaries
Understanding sporadic Alzheimer’s (sAD) requires understanding apolipoprotein E (APOE). No gene determines risk for sAD to a greater extent than Apoe. As such, contributions of APOE to AD are intensively studied, to the point that multiple sessions at AAIC 2023 were dedicated to it. Here, we cover several of the most important presentations on this sAD risk factor.
APOE as an export protein
For neurons, APOE is largely viewed as an import protein, delivering cholesterol and other lipids. According to work shared by Femke Feringa (VU Amsterdam) on the effects of APOE variants on lipid metabolism, it also serves as an export protein, helping neurons to clear toxic fatty acids. She demonstrated the ability for neurons to export lipids to both astrocytes and microglia by providing neurons with fluorescently-labeled lipids, which were trafficked to glia subsequently added to the culture. She then found that human astrocytes form significantly more lipid droplets (LDs) when co-cultured with human neurons expressing APOE3 than when cultured alone. When neurons expressing APOE4 are added to the culture, however, no such increase occurs. Exporting fatty acids from neurons, which cannot break them down, to glia that can metabolize them prevents these lipids from becoming peroxidized and damaging cellular structures. Thus, one element of how APOE4 confers AD risk may involve the accumulation of toxic fatty acids due to impaired clearance mechanisms.
While recent studies have found that murine neurons export lipids to murine glia in an APOE genotype-dependent manner, this is the first report of it also being observed with human cells.
APOE genotype modifies microglial immunometabolism
APOE variants determine how mouse astrocytes metabolize glucose, with APOE4 causing them to favor aerobic glycolysis over mitochondrial respiration. For Nicholas Devanney (University of Kentucky), this was reminiscent of changes observed in immune cells; pro-inflammatory signals trigger a switch to glycolysis driven by the transcription factor Hif1a, whereas anti-inflammatory pathways require increased mitochondrial respiration. Since microglia sit at the intersection between glia and immune cells, he speculated that the ability for APOE4 to induce pro-inflammatory phenotypes in microglia could be due to shifts in metabolism.
Using single-cell RNA-Seq, he found that APOE4 affected expression of metabolic genes across all glial types, including microglia. Fewer of these microglia expressed markers of the homeostatic phenotype, while more of them expressed markers of the “disease-associated microglia” (DAM) phenotype. These DAM-like cells were the only microglia to show increased expression of APOE during aging. Like other pro-inflammatory immune cells, this cluster of microglia showed the highest expression of Hif1a and glycolytic genes, with metabolic profiling largely finding that they resembled pro-inflammatory macrophages. One exception, however, is itaconate, which is made from citric acid cycle intermediates and induces anti-inflammatory pathways. While itaconate levels increase in pro-inflammatory macrophages, likely keeping inflammation within an appropriate range, itaconate levels are reduced in APOE4 microglia.
These results suggest that metabolic changes within microglia could contribute to their role in promoting inflammation and neurodegeneration, a hypothesis Devanney terms “immunometabolism.”
APOE4 prevents microglial activation
While chronic inflammation and immune activation are harmful in AD, it does not necessarily follow that completely suppressing these events will prove therapeutic. Normal microglial function, after all, requires them to occur transiently. Oleg Butovsky (Brigham and Women’s Hospital) outlined how APOE4 can have a negative impact by preventing microglial activation.
Using an approach where apoptotic neurons are injected into the mouse brain to activate DAMs and cause neurodegeneration, he found that APOE4 reduced acute DAM activation. Deleting APOE4 from microglia in both the tau P301S mouse model and the “Iberian mutation plus presenilin-1 L166P” (APP/PS1) model promoted the DAM phenotype, showing reduced neural loss and increased plaque clearance. RNA sequencing of human brain tissue found that APOE4 was associated with increased levels of “checkpoint” proteins in the transforming growth factor-β (TGF-β) signaling pathway, with this being most prominent in female samples. He further found that blocking integrin beta subunit 8 (ITGB8), released by astrocytes to promote TGF-β signals in microglia, could promote the DAM phenotype and reduce neural loss in the APP/PS1 mouse model.
Combined with Devanney’s research, these results indicate that APOE4 presents a dual threat to microglia. It weakens their ability to activate for clearing amyloid plaques and apoptotic cells, promoting neurodegeneration. Those that do get activated, however, become unable to return to homeostasis, causing additional harmful effects to surrounding tissue.
Could editing APOE4 later in life reduce AD risk?
The extreme risks associated with APOE4 have made it an attractive target for gene therapies. Numerous groups have attempted to reduce APOE4 levels or provide compensatory APOE2. However, while a recent preprint argues that loss of one APOE4 allele is not harmful, complete loss of APOE4 has drawbacks. Supplementation with APOE2, meanwhile, still allows for existing APOE4 to exert its toxic gain-of-function effects.
What if genomic editing replaced APOE4 with APOE2? Could this improve outcomes for patients, even if performed later in life? These questions were explored in a pair of talks by Lesley Golden and Lance Johnson (University of Kentucky), with promising results.
In their model, mice have a special APOE4 knock-in allele that “switches” to APOE2 upon Cre recombination (“switch allele”). This strategy is robustly effective at switching the APOE allele, achieving >90% efficiency. A full-body switch spiked plasma triglyceride levels; since this is a phenotype observed in human APOE2 carriers, this is both a drawback to the approach and a sign that it is switching the allele effectively. They then used Cre lines that would allow for cell type-specific manipulations, both to avoid altering blood lipid levels and to see which cells might provide a benefit when switched.
Using the 5xFAD mouse model as a background, they crossed in the switch allele and Aldh1l1-CreERT2, which drives Cre expression in astrocytes following tamoxifen administration. They induced the switch at 6 months of age – at which point significant amyloidosis has already occurred – and studied the mice 2 months later. Switching to APOE2 in astrocytes was sufficient to provide robust improvements, with “switched” mice showing lower levels of amyloid buildup, reactive microglia, and reactive astrocytes, as well as improved memory on an associated fear conditioning task.
The results from performing the switch in microglia were less promising. While plaque load and gliosis are trending downward in their currently limited sample size, they found no improvement on the fear conditioning task, indicating minimal cognitive benefit. In fact, they found that levels of reactive microglia increased following the switch, whereas switching in astrocytes reduced reactive microglia levels. These results somewhat contrast with prior studies finding, for example, that APOE4 microglia secrete neurotoxic factors. Since the switch is occurring in a background of APOE4 astrocytes, one possibility is that astrocytes are the dominant contributors to APOE-related phenotypes, since astrocytes generate the majority of the brain’s APOE. In this scenario, APOE4 astrocytes obscure any benefits from switching microglia. Another possibility is that 6 months of age is too late to gain improvements from switching microglia, even if it is a viable timepoint for astrocytes.
Regardless, the results observed after “switching” astrocytes suggests that genetic editing strategies could provide therapeutic benefits, even after pathology has already started taking hold.
Conclusion
These talks discussed some of the numerous, complex ways in which APOE influences AD etiology. Some shed new light on established mechanisms, such as effects on glial metabolism, while others touched on pathways that are just beginning to be understood. The gene therapies explored by the team at the University of Kentucky provide hope that editing APOE4 could curb the high risk for AD in those that carry it.